Secret dimensions
In everyday life, we inhabit a space of three dimensions – a vast ‘cupboard’ with height, width and depth, well known for centuries. Less obviously, we can consider time as an additional, fourth dimension, as Einstein famously revealed. But just as we are becoming more used to the idea of four dimensions, some theorists have made predictions wilder than even Einstein had imagined.String theory intriguingly suggests that six more dimensions exist, but are somehow hidden from our senses. They could be all around us, but curled up to be so tiny that we have never realized their existence.
Beyond the third dimension
Some string theorists have taken this idea further to explain a mystery of gravity that has perplexed physicists for some time – why is gravity so much weaker than the other fundamental forces? Does its carrier, the graviton, exist and where? The idea is that we do not feel gravity’s full effect in the everyday world. Gravity may appear weak only because its force is being shared with other spatial dimensions.To find out whether these ideas are just products of wild imaginations or an incredible leap in understanding will require experimental evidence. But how?
High-energy experiments could prise open the inconspicuous dimensions just enough to allow particles to move between the normal 3D world and other dimensions. This could be manifest in the sudden disappearance of a particle into a hidden dimension, or the unexpected appearance of a particle in an experiment. Who knows where such a discovery could lead!
ALICE
A Large Ion Collider Experiment
For the ALICE experiment, the LHC will collide lead ions to recreate the conditions just after the Big Bang under laboratory conditions. The data obtained will allow physicists to study a state of matter known as quark‑gluon plasma, which is believed to have existed soon after the Big Bang.All ordinary matter in today’s Universe is made up of atoms. Each atom contains a nucleus composed of protons and neutrons, surrounded by a cloud of electrons. Protons and neutrons are in turn made of quarks which are bound together by other particles called gluons. This incredibly strong bond means that isolated quarks have never been found.
Collisions in the LHC will generate temperatures more than 100,000 times hotter than the heart of the Sun. Physicists hope that under these conditions, the protons and neutrons will "melt", freeing the quarks from their bonds with the gluons. This should create a state of matter called quark-gluon plasma, which probably existed just after the Big Bang when the Universe was still extremely hot. The ALICE collaboration plans to study the quark-gluon plasma as it expands and cools, observing how it progressively gives rise to the particles that constitute the matter of our Universe today.
A collaboration of more than 1000 scientists from 105 physics institutes in 30 countries works on the ALICE experiment (November 2011).
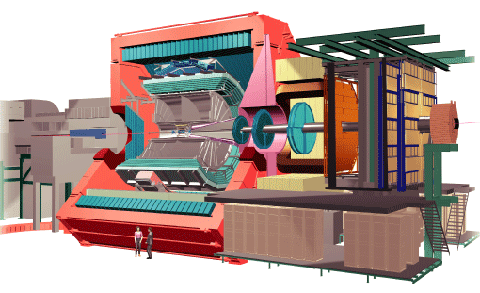
ALICE detector
- Size: 26m long, 16m high, 16m wide
- Weight: 10,000 tonnes
- Design: central barrel plus single-arm-forward muon spectrometer
- Location: St Genis-Pouilly, France. See ALICE in Google Earth.
Related links
- The ALICE website
-
LHCb
Large Hadron Collider beauty
The LHCb experiment will help us to understand why we live in a Universe that appears to be composed almost entirely of matter, but no antimatter.
It specialises in investigating the slight differences between matter and antimatter by studying a type of particle called the 'beauty quark', or 'b quark'.
Instead of surrounding the entire collision point with an enclosed detector, the LHCb experiment uses a series of sub-detectors to detect mainly forward particles. The first sub-detector is mounted close to the collision point, while the next ones stand one behind the other, over a length of 20 m.
An abundance of different types of quark will be created by the LHC before they decay quickly into other forms. To catch the b-quarks, LHCb has developed sophisticated movable tracking detectors close to the path of the beams circling in the LHC.
The LHCb collaboration has 650 scientists from 48 institutes in 13 countries (April 2006).
LHCb detector
- Size: 21m long, 10m high and 13m wide
- Weight: 5600 tonnes
- Design: forward spectrometer with planar detectors
- Location: Ferney-Voltaire, France.
Related links
- The LHCb website
-
TOTEM
TOTal Elastic and diffractive cross section Measurement
The TOTEM experiment studies forward particles to focus on physics that is not accessible to the general-purpose experiments. Among a range of studies, it will measure, in effect, the size of the proton and also monitor accurately the LHC's luminosity.
To do this TOTEM must be able to detect particles produced very close to the LHC beams. It will include detectors housed in specially designed vacuum chambers called 'Roman pots', which are connected to the beam pipes in the LHC. Eight Roman pots will be placed in pairs at four locations near the collision point of the CMS experiment.
Although the two experiments are scientifically independent, TOTEM will complement the results obtained by the CMS detector and by the other LHC experiments overall.
The TOTEM experiment involves 50 scientists from 10 institutes in 8 countries (2006).TOTEM detector
- Size: 440 m long, 5 m high and 5 m wide
- Weight: 20 tonnes
- Design: Roman pot and GEM detectors and cathode strip chambers
- Location: Cessy, France (near CMS)
Related links
LHCf
Large Hadron Collider forward
The LHCf experiment uses forward particles created inside the LHC as a source to simulate cosmic rays in laboratory conditions.
Cosmic rays are naturally occurring charged particles from outer space that constantly bombard the Earth's atmosphere. They collide with nuclei in the upper atmosphere, leading to a cascade of particles that reaches ground level.
Studying how collisions inside the LHC cause similar cascades of particles will help scientists to interpret and calibrate large-scale cosmic-ray experiments that can cover thousands of kilometres.
The LHCf experiment involves 22 scientists from 10 institutes in 4 countries (September 2006).
LHCf detector
- Size: two detectors, each measures 30 cm long, 80 cm high, 10 cm wide
- Weight: 40 kg each
- Location: Meyrin, Switzerland (near ATLAS)
No comments:
Post a Comment